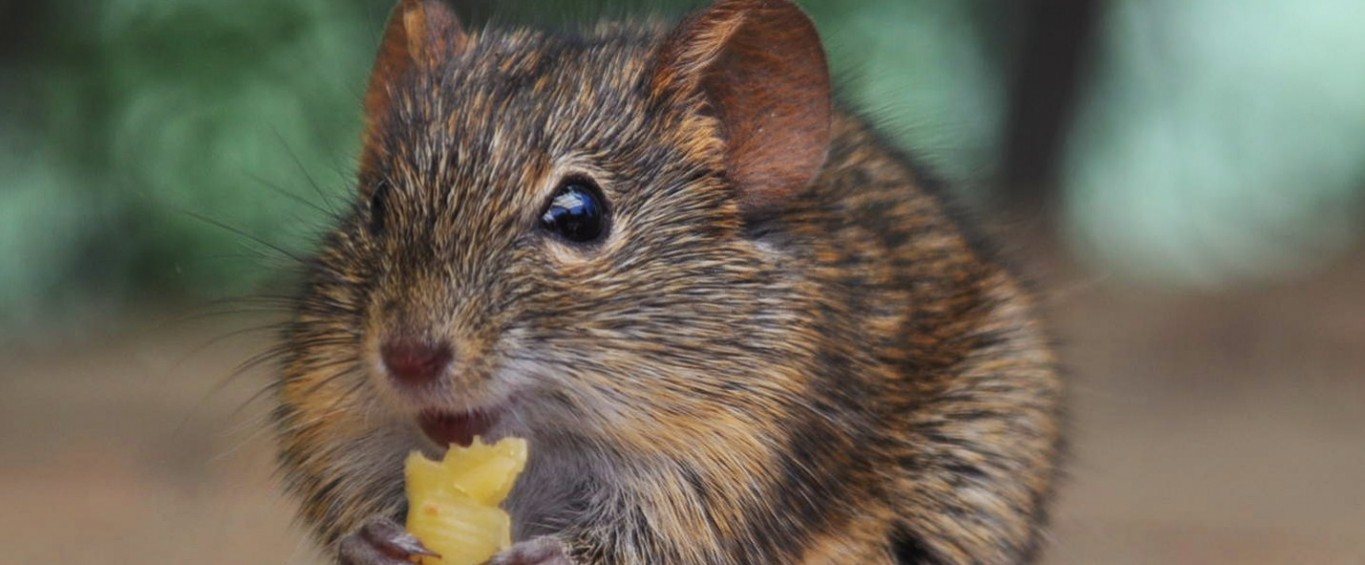

Of Mice and Smells
A new study carried out by researchers at the Collège de France has shown that the brains of mice are capable of compensating for limited olfactory information in order to maintain a normal olfactory perception.
Sensory Perception
Sensory perception functions by the transformation of stimuli, detected by the sensory organs, into patterns of neuronal activity in the brain. These patterns form an internal representation of the stimuli of the exterior world. They constitute the neurobiological basis of perception and behaviours that depend on sensory experience.
A study led by Benjamin Roland, part of Dr. Fleischmann’s team at the Collège de France, together with researchers at the Francis Crick Institute in London, has shed light upon the mechanisms at work in this transformation that is integral to perception.
The researchers studied the capacity of the olfactory system of mice (Figure 1) to process very weak sensory signals. Their findings show that the mouse’s brain is capable of compensating for faint signals in order to regenerate useable information.
Figure 1. The olfactory system
In the nose, odours are detected by olfactory receptors at the surface of the neurons which are located in the olfactory epithelium.
Olfactory information is then transmitted to the brain, and more precisely to the olfactory bulb, where an initial representation of odours is formed.
These cells are connected in turn to the olfactory cortex, which processes olfactory information at a higher level. The olfactory cortex plays in integral role in the recognition of odours and in the processes of the olfactory memory.
Compensation Mechanisms
"The olfactory epithelium of the mouse consists of around 1300 different olfactory receptors, which allow for the recognition of an infinite combinations of odour molecules," explains Dr. Fleischmann, who headed up the study. "Eight years ago, we modified this structure, using a process of transgenesis to create a mouse whose nose was ‘monoclonal.’ In these monoclonal mice, 95% of the olfactory receptors were identical, and their olfactory differentiation was therefore reduced to one-twentieth of its original capacity."
The images below show the contrast between the functioning of the ‘natural’ nose in a control group, and that of the nose of the transgenic mice, where so-called ‘M71’ receptors account for 95% of the total olfactory receptors.
Figure 2. Modification of the sensory map in ‘monoclonal’ nose of transgenic mice.
To obtain the images on the left, the researchers used a marker in order to show the distribution of sensors in the olfactory epithelium. The top image shows in red the distribution of M71 receptors in normal mice: it is present only in a small fraction of sensory neurons. In the lower image, however, the predominance of red shows that the M71 receptor is omnipresent in the transgenic mice.
The images on the right show the activity of the olfactory epithelium in response to different odours (ethyl acetate, eugenol, and amyl acetate). This activity is revealed using a fluorescent marker.
The control mice show a normal level of activity in the olfactory epithelium.
In the transgenic mice, where the M71 receptor dominates, these odours fail to activate the receptor and thus no neuronal activity is produced.
However, despite this modification, the transgenic mice are nonetheless able to detect a wide variety of olfactory stimuli, including odours which do not activate the M71 receptor. "We drew up the hypothesis in 2008 that the neuronal circuits of the olfactory bulb are capable of amplifying very weak sensory signals from the nose to regenerate more complete sensory information," said Dr. Fleischmann.
The Experiment and its Implications
To test this hypothesis, the researchers at the Collège de France and at the Francis Crick Institute observed the activity of the neurons in the mouse’s olfactory bulb in response to odours; to do so, they used imaging techniques and in vivo electrophysiology.
"Electrophysiology allows us to directly measure the electric activity of a single neuron over a given period of time," explained Benjamin Roland. "The in vivo imaging that we use in our laboratories is less precise in terms of time, but makes it possible to follow the activity of several dozen neurons at once" (Figure 3).
Figure 3. In vivo imaging of neuronal activity.
Neuronal activity (animated image, centre) is tracked by measuring the changes in fluorescence in groups of cells in the olfactory bulb as the mouse is exposed to an odour (left). When a neuron reacts to this stimulation, its fluorescence increases.
Using this technique the researchers showed that despite the absence of neuronal activity in the olfactory epithelium in transgenic mice, the patterns of neuronal activity in the olfactory bulb were for generally normal: although there is no neuronal activity in the olfactory epithelium in the M71 mice, the odours activate the same number of cells in the olfactory bulb as they do in the control mice.
This ‘normalisation’ of neuronal activity enables olfactory circuits to amplify very weak sensory signals, or, conversely, to reduce excessively intense signals.
For Dr. Fleischmann, this observation is consistent with their initial hypothesis: "The brain of the transgenic M71 mice compensates for the deficient nose to enable them to detect the majority of odours in their environment."
The implications of the study and its validation of the team’s original hypothesis open up a number of interesting perspectives for research into sensory perception and its consequences for behaviour. The article containing the researchers’ findings has been published in e-Life (see below for complete reference).
Glossary
Transgenesis: a technique in genetic engineering through which scientists can modify the genetic makeup of an animal by introducing one or several fragments of DNA. The process makes it possible to modify the expression of genes or to introduce entirely new ones into the cells of an organism.
Electrophysiology: refers to the techniques with which it is possible to measure the electrical activity of a neuron.
In vivo imaging: technology which allows for the observation of the activity of large groups of neurons in a living animal. Through a process of transgenesis, it is possible to mark selected neurons of interest to researchers with a fluorescent molecule. When a neuron is active, an influx of calcium ions causes the fluorescence to increase. By measuring the changes in neurons’ fluorescence over time, it is possible to create spatial maps of neuronal activity in response to stimuli.
References
Roland, B., Jordan, R., Sosulski, D.L., Diodato, A., Fukunaga, I., Wickersham, I., Franks, K.M., Schaefer, A.T., and Fleischmann, A. (2016). Massive normalization of olfactory bulb output in mice with a "monoclonal nose." eLife 5.
Fleischmann, A., Shykind, B.M., Sosulski, D.L., Franks, K.M., Glinka, M.E., Mei, D.F., Sun, Y., Kirkland, J., Mendelsohn, M., Albers, M.W., Axel, R., 2008. Mice with a "monoclonal nose": perturbations in an olfactory map impair odor discrimination. Neuron 60, 1068–81.